Mastering Thin Film Hydration Method for Liposome and Lipid Nanoparticle Formulation
Thin Film Hydration, the genesis of liposomes and Lipid nanoparticles
The Thin Film Hydration Method is a cornerstone in the non-viral drug delivery field as it was the first available method offering the ability to fabricate liposomes or other lipid-based nanoparticles such as lipid nanoparticles (LNP) for targeted drug delivery. Popular for its ease of use and efficacy in encapsulating diverse compounds - hydrophilic or hydrophobic API, small drugs, peptides, proteins, and RNA - this synthesis technique, also known as the Bangham method or Lipid Film Hydration Method, has revolutionized approaches in drug delivery.
Liposomes and lipid nanoparticles, stand out as versatile carriers of submicron size (generally 50 to 500 nm). Liposomes are composed of one or more hollow phospholipid bilayer shells entrapping aqueous vesicles, whereas lipid nanoparticles (LNPs) are characterized by a solid lipid matrix. This unique construction provides them with unique capabilities for entrapping Active Pharmaceutical Ingredients (APIs), such as drugs for liposomes, or oligonucleotides, RNA, and DNA… for the LNP. Notably, they offer excellent biocompatibility, high encapsulation efficiency, and good drug release capability, making them a key asset for targeted drug delivery.
This method has gained broad popularity in many laboratories due to its ease of use, widespread availability, and cost-effectiveness. However, poor scalability and difficulty in achieving consistent control over the final nanoparticle characteristics tend to be challenging and often lack reproducibility, which can result in suboptimal performance for biological applications.
For this reason, the thin film hydration process has been continuously improved over the years leading to novel nanoparticle synthesis methods, offering much finer control of the nanoparticle outcome while allowing for higher throughput. These innovations are making the latest generation of liposomes and LNP an ideal tool for targeted delivery or theranostic application
Preparation of Liposome or Lipid-based nanoparticle by thin-Film Hydration Method
The process of Liposome preparation by Thin Film Hydration involves dissolving lipids in organic solvents, creating a thin lipid film in a flask by solvent evaporation, and hydrating this film with aqueous solutions to trigger the nanoparticle formation. Numerous critical factors are influencing this process including the choice of lipids, solvent types, and hydration parameters. Let's dive into its step-by-step process to better understand it!
Selection of Lipids
The first step of the process, and one of the most critical, involved choosing the appropriate lipids for your liposome formulation. Indeed, their choice drives nearly all the final nanoparticle characteristics, from size, and drug encapsulation efficiency (thus drug content) to cellular uptake and biodistribution. Common liposomal formulation involves soy phosphatidylcholine (SPC), cholesterol (Chol), PEG (polyethylene glycol), and other specific lipids depending on the application…
The proportion of lipids is critically significant, as exemplified by PEG. Its concentration typically ranges from 0.5 to 3%, and this variation can almost divide by three the size of nanoparticles or liposomes.
Dissolution of Lipids in an organic solvent
The second step involves the dissolution of lipids in an organic solvent, typically chloroform or a chloroform-methanol mixture, to obtain a lipid suspension. Nevertheless, less harsh solvents such as pure ethanol or methanol can be used. Typically, a round-bottom flask should be used for this purpose, as it eases the next step.
Lipophilic API and hydrophobic drug can also be dissolved into the solvent at that stage.
Formation of Thin Lipid Film
Once dissolved, the lipid-solvent mix should be transferred to a rotary evaporator. Using it, the organic solvent will be fully evaporated under reduced pressure to form a thin film of lipids at the bottom of the flask. Following evaporation, the thin film should be dried under vacuum for several hours to remove any residual solvent.
Hydration of Lipid Film
The next step of the process involves the hydration of the dried lipid film with a suitable aqueous phase, such as distilled water, buffer solution, or saline containing the hydrophilic API. The hydration medium can also be preheated when using lipids with a high phase-transition temperature (Tc).
Putting in contact the lipids with the aqueous phase will trigger the self assembly process of the liposome, leading to the formation of multilamellar vesicles (MLVs).
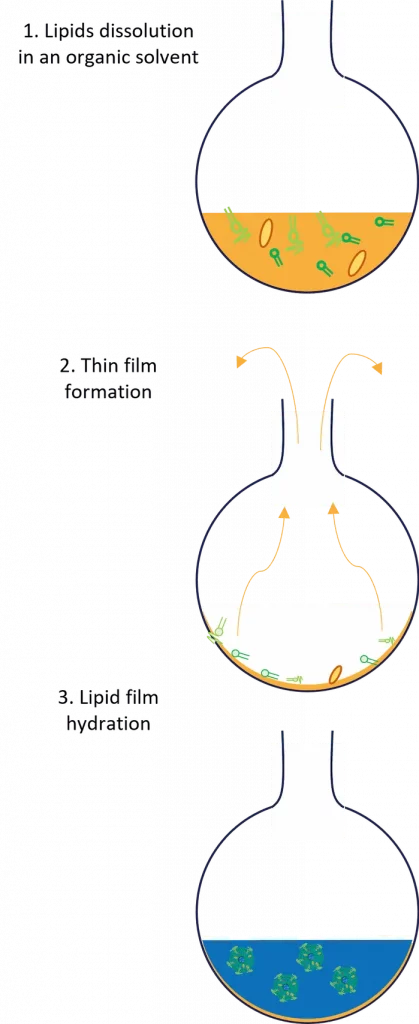
Post-processing step: Purification
Following the formation process, substances like unencapsulated drugs and contaminants often remain in the surrounding liquid. Another key element of post-production involves removing any leftover organic solvents used in the process. Indeed, if these solvents linger in the final product, they can destabilize the liposomes and be risky for use. 1
These substances must therefore be removed in an additional purification step. Common techniques to remove them include tengential flow filtration, ultra-centrifugation, dialysis. However, these purification processes can be time-consuming and may significantly impact the final nanoparticle characteristics while reducing the final yield of liposomes.
Characterization of the liposome product
As described in our review on LNP and Liposome characterization, numerous parameters such as particle size, polydispersity, surface charge, drug loading, and encapsulation efficiency are affected by the LNP manufacturing method. Advanced analytical techniques such as DLS are employed to measure these attributes, ensuring the quality and applicability of the liposomes and LNPs for biological and pharmaceutical applications.
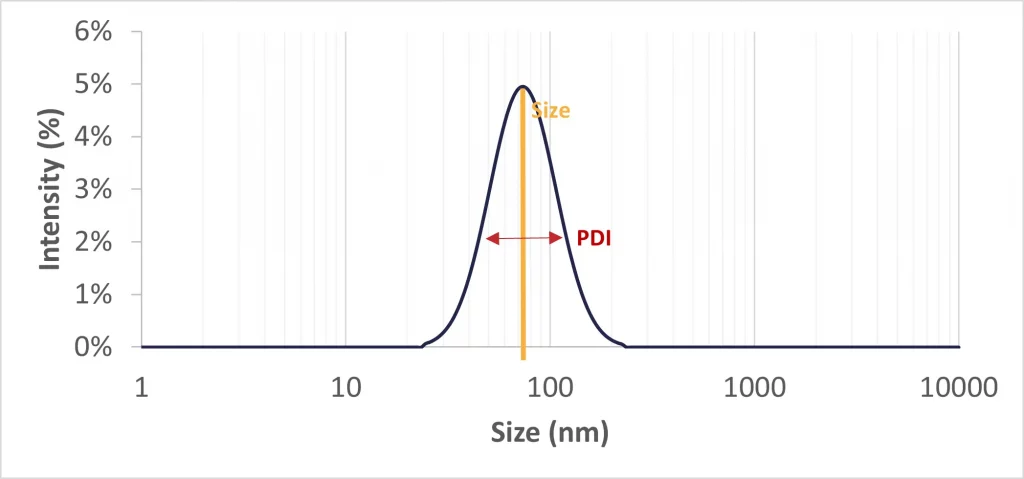
Nanoparticle size
Generally, nanoparticles vary in size from 50 nm to 500 nm, but an optimal size target falls within the 50 to 150 nm range. This size range is preferred as it enhances cellular uptake and evades immune system clearance, thereby ensuring an extended circulation time in the bloodstream. As mentionned above, particle size is generally affected by lipid ratios and concentration. Nanoparticles and liposomes obtained using thin film hydration technique tend to be rather large (several 100s µm) and thus require extra post refining steps.
Polydispersity Index
The Polydispersity Index (PDI) measures the uniformity of a liposomal population. As per FDA guidelines, the PDI for a pharmaceutical drug product should be maintained below 0.3. This presents a significant limitation of the lipid hydration method, which typically yields a PDI ranging between 0.3 to 0.5. So, additional refinement steps, such as extrusion, can be needed to meet these standards.
Zeta potential
The liposome zeta potential is a measure of the charge near the surface of the liposome structure. The Zeta potential affect both the nanoparticle stability and toxicity, as a low Zeta potential means a low stability and risks of aggreration, while too high values of zeta potential lead to toxicity. It is generally measured using a Zeta sizer, and its value should remain between -30 and 30 mV. The main parameter affecting the Zeta potential is the nanoparticle composition.
Structure and morphology
Liposomes can take variable forms such depending on the number of vesicules surrounded by a lipid bilayer they contain. These variations include unilamellar and multilamellar vesicles (MLVs), which present an onion like structure, each distinguished by their unique arrangement and composition. For precise characterization of morphology, Transmission Electron Microscopy (TEM) or its improved version, the Cryo-TEM, is employed as an essential and highly effective tool.
Encapsulation efficiency
The encapsulation efficiency, or entrapment efficiency, indicates the ratio of loaded drugs over the initial amount of drug initially available. It is therefore highly dependent on the characteristics of the used drugs, and the lipid composition.
Pro and cons of the Banger method
The advantages and drawbacks of the Banger methods are summarized as follows:
Advantages:
- Ease of Access and Cost-Effectiveness: The Banger method primarily utilizes standard laboratory equipment, which is readily available in most labs, making it an accessible and cost-efficient approach for liposome formulation.
- Reduced Solvent Contact: As the organic solvent is almost entirely removed upon encountering the aqueous solution, hydrophilic Active Pharmaceutical Ingredients (APIs) or drug products are minimally exposed to the solvent
Drawbacks and Limitations:
- Challenges in Solvent Removal: Complete elimination of the organic solvent can be tedious, leading to a very long synthesis time.
- Colloidal Stability and Entrapment Efficiency: The method often results in low colloidal stability and variable entrapment efficiency, leading to quality issues with the final drug product.
- Scale Limitations: While suitable for small-scale production, the method is time-consuming and incurs additional costs when scaled up for larger production due to the low volume per batch and long process duration.
- Poor repeatability: Due to the lack of control of the mixing parameters during the self-assembly process, the batch-to-batch repeatability is very poor, again leading to quality limitations for pharmaceutical applications.
These issues can impact the clinical efficacy of the resulting nanoparticles. Recent innovations in the field aim to optimize this method, enhancing the quality and functionality of the nanoparticles.
How to improve the quality of liposomes produced by thin film hydration?
Numerous approaches can be used to improve the quality of the final liposomal formulation
Extra post-processing steps
Such as for other conventional liposome preparation methods, including solvent injection methods (ethanol injection, Ether injection…) or detergent removal methods… extra downsizing or post process steps can be carried out to improve the quality of the liposome drug product. While different in practice, all these methods employs the same approach to decrease the nanoparticle size, and homogenize the nanoparticle population: they involve the use of high energy, to break down the nanoparticle into smaller more uniform particles.
Sonication Method
The sonication method employs ultrasonic energy to create smaller vesicles from multilamellar liposome (MLV) solutions, typically conducted under an inert atmosphere. Two technics can be used for this purpose: bath sonication and probe sonication.
Probe Sonication
Probe sonication involves immersing a sonicator tip into the solution, making it simple to use and ideal for small volumes. Yet, main disadvantages include potential metal contamination, and required cooling to prevent lipid degradation.
Bath Sonication
When increasing scale, bath sonication bath sonication in a temperature controlled environment becomes to prefered synthesis method. While effieincy, this method lead to low encapsulation efficiency, potential degradation and tend to increase polydispersity.
To conclude on sonication, despite its popularity for producing smaller liposomes, it may not be ideal for liposomes requiring precise physical properties such as for biological applications.
Extrusion Method
Extrusion method involves forcing liposome solutions through membranes with specific pore sizes, often performed above the phospholipids' phase-transition temperature. This results in liposomes size reduction with narrow size distributions.
Known for producing consistent and reproducible liposome population, the extrusion method also exhibits major limitation such as: product loss, limited working volumes and high pressure and temperautre involved, altering the API quality.
High-Pressure Homogenization Method
In the high-pressure homogenization process, initial liposome suspensions are subjected to intense pressure. This high-pressure environment causes the liposomes population to downsize, a result of cavitation, shear forces, and turbulence within the suspension. The characteristics of the resulting liposomes are influenced by several key factors, including the applied pressure, the temperature during processing, and the number process cycles.
However, this technique has its challenges. One of the primary concerns is the requirement for extremely high pressures, which can be demanding to achieve and maintain. Additionally, there's a risk of contamination from metal and oil, which can compromise the purity and quality of the liposomes.
In addition to these refinement process, cutting-edge synthesis liposome and lipid-based nanoparticle synthesis methods have been developed to overcoming the method's traditional limitations, paving the way for more effective drug delivery systems.
Microfluidics: A Cutting-Edge Approach Surpassing Thin Film Hydration for Liposome and LNP Synthesis
Microfluidics has emerged as a superior alternative compared to the traditional approach for Liposomes and Lipid Nanoparticle Formulation. This state-of-the-art technology offers unparalleled control over particle size, reduced variability, higher encapsulation efficiency, and better scalability. This positions microfluidics as the most advanced and efficient method in nanoparticle fabrication.
Limitations of the current methods for the control of nanoparticle characteristics
Conventional liposome production methods typically involve mixing a lipid-containing solvent with an anti-solvent, usually water, which contains the Active Pharmaceutical Ingredient (API). The interaction between these two solutions triggers nanoparticle synthesis through a self-assembly process, also known as nanoprecipitation. Consequently, the mixing conditions significantly influence the final nanoparticle characteristics. Achieving consistent mixing and reproducibility, both between batches and within a single sample, is crucial for obtaining a homogeneous nanoparticle population with optimal size control and batch-to-batch consistency.
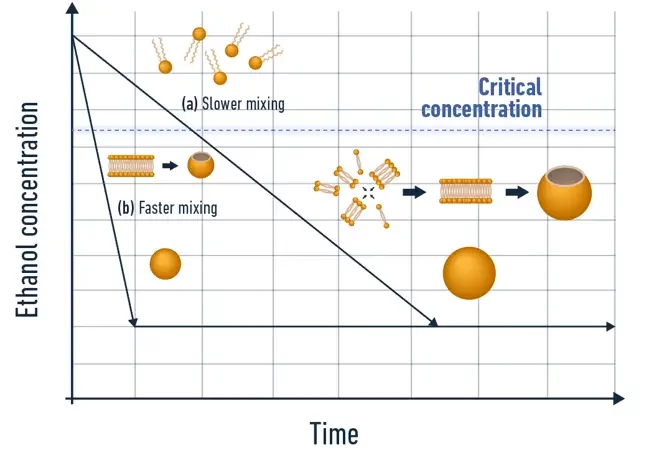
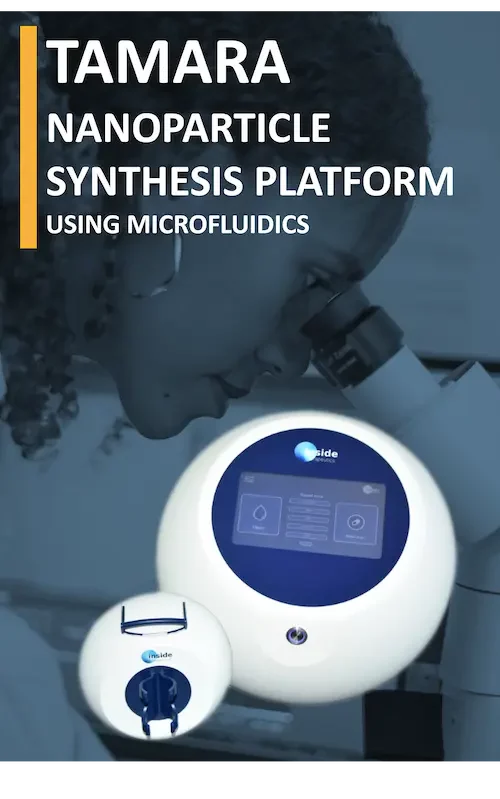
Introduction to nanoparticle synthesis by microfluidics
Microfluidics, the science of manipulating liquids at a sub-millimeter scale, emerges as a pivotal tool in this context. At these scales, fluid exhibits laminar flow properties, enabling highly controlled and reproducible fluid conditions. This predictability is key to consistently controlling the mixing conditions, thereby allowing precise determination of the final nanoparticle characteristics. Notably, microfluidics not only improves control over the final product but also offers high throughput (up to tens of mL/min), leading to significantly reduced operation times (under 1 minute per batch), greatly accelerating the screening process.
Characteristics of nanoparticles obtained with microfluidics
Microfluidics enhance control and quality across all critical nanoparticle attributes:
Size and PDI: Superior mixing control ensures a homogenous nanoparticle population (PDI <0.2), excellent size control, and remarkable batch-to-batch repeatability (better than 5%), making it ideal for pharmaceutical nanoparticle synthesis.
Encapsulation Efficiency: Microfluidics achieves unparalleled encapsulation efficiency, reaching up to 98% in RNA-LNP encapsulation, for example.
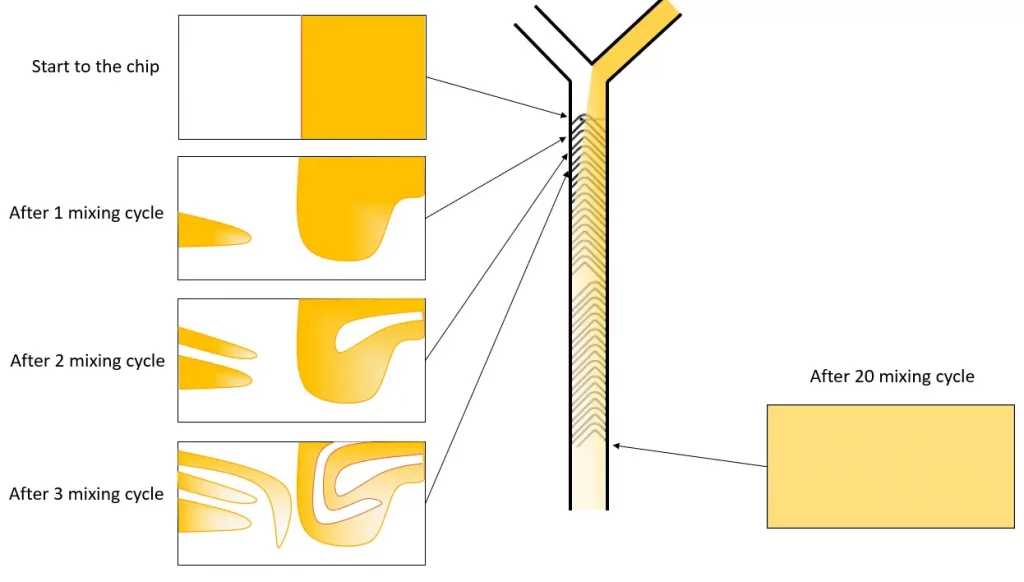
Practical Implementation
Microfluidic systems typically consist of two main components: a microfluidic chip with channels ranging from 5 to 500 µm in diameter, and flow control systems such as syringe pumps or advanced pressure-driven systems. The design of the microfluidic chip, particularly advanced designs like the herringbone mixer, directly influences the mixing conditions, allowing for reproducibility, high-speed synthesis, and straightforward control of particle size by adjusting the flow rate.
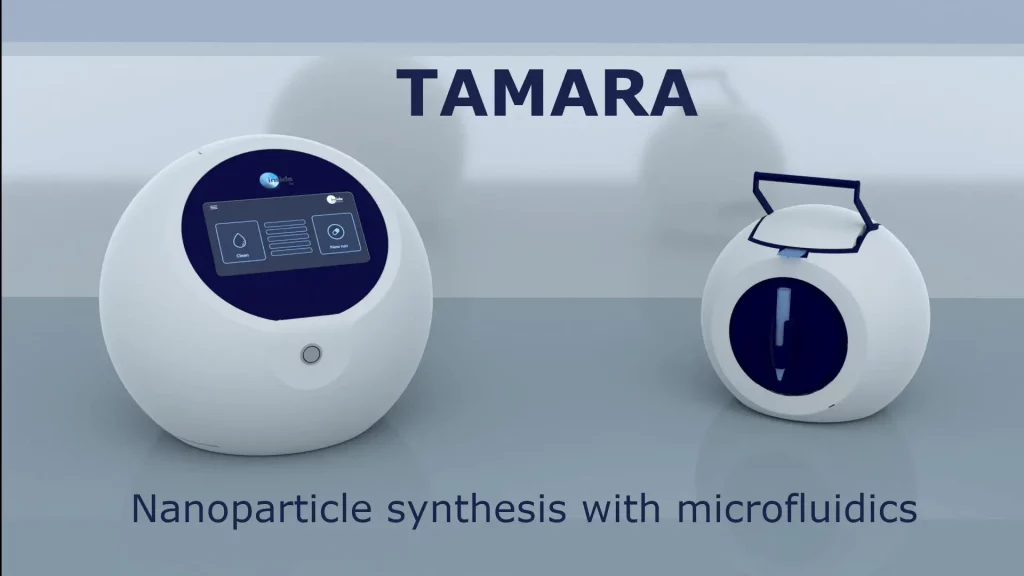
Despite its benefits, microfluidics can be complex due to the specificity of its components and the precision required for nanoparticle production, especially at sub-mililiter volumes.
To address this, we have launched the TAMARA platform which offers a user-friendly solution, simplifying the application of microfluidics in nanoparticle synthesis, even for begginers. For more information, visit TAMARA Platform.
In conclusion, microfluidics represents a paradigm shift in nanoparticle production, offering significant improvements over traditional methods. Its precision, reproducibility, speedand scalability make it an invaluable asset in the field of pharmaceuticals and nanotechnology."
The Historical Significance of Thin Film Hydration in Liposome and Lipid Nanoparticle Applications
The emergence of liposomes, originally formulated through the thin film hydration method, marked a revolutionary shift in the landscape of drug delivery and biomedical research. Originating from the pioneering work of Alec Bangham in the 1960s, this method set the standard for liposome production. It serves as a historic milestone, demonstrating how a single scientific advancement can open a plethora of doors in medical science and pharmaceutical applications.
Foundation in Drug Delivery
Thin film hydration introduced a revolutionary approach to encapsulating both hydrophilic and hydrophobic drugs within lipid bilayers. This innovation propelled the field of targeted drug delivery forward, offering new therapeutic possibilities for a variety of health conditions.
Cancer Therapy Breakthroughs
The method facilitated the development of liposomal drug formulations specifically designed for cancer treatment. By encapsulating chemotherapeutic agents within liposomes, and leveraging on the EPR effect, researchers achieved targeted tumor delivery, significantly reducing the systemic toxicity and side effects associated with regular cancer chemotherapy. A prime example is the first liposomal formulation of the cytotoxic anticancer agent, doxorubicin or doxyl.
Advancements in Genetic Medicine
The progression in liposome research, coupled with improvement in the synthesis techniques of lipid-based nanoparticles, such as microfluidics, lead to the development of Lipid Nanoparticles (LNPs). These LNPs, close cousin to liposomes, are specifically tailored to encapsulate genetic materials such as DNA, RNA, or siRNA.
They opened up doors to groundbreaking solutions for correcting genetic disorders, particularly in the development of treatments for rare diseases.
Immunization and Vaccine Development
Historically, liposomes formulated through thin film hydration methods have played a crucial role in vaccine formulation. Their ability to enhance immune responses and provide targeted delivery of antigens has been instrumental in the development of various vaccines. This culminated with the use of LNP to protected the mRNA in the COVID-19 vaccine.
Cosmetic Applications
The cosmetic industry harnessed the potential of liposomes for skin treatment and rejuvenation. By delivering active ingredients directly to targeted skin layers, liposomes improved the efficacy of skincare products and treatments. Due to the lower regulatory constrains linked to cosmetic applications, thin film hydration methods is still widely used in this field.
Conclusion and Future Perspective on thin film hydration
In summary, the thin film hydration method not only laid the foundation for liposome development, but also led to advancements in the broader field of lipid-based nanoparticles. However, the limited control over critical nanoparticle attributes inherent in this method poses limitations for its application in biological and pharmaceutical field. Neverthless, this approach led to the development of advanced liposome production techniques, particularly the emergence of microfluidics. This played a crucial role in the discovery of Lipid Nanoparticles (LNPs), which has been a cornerstone in initiating a new era of RNA-based research, paving the way for novel cancer, infectious disease and rare disease treatement. Even with limitations, the thin film hydration technique's historical impact still drives current research, highlighting its enduring role in healthcare and science.
Interested in learning more about nanoparticle synthesis?
References
1 Lombardo, Domenico, and Mikhail A Kiselev. “Methods of Liposomes Preparation: Formation and Control Factors of Versatile Nanocarriers for Biomedical and Nanomedicine Application.” Pharmaceutics vol. 14,3 543. 28 Feb. 2022, doi:10.3390/pharmaceutics14030543
2 Umbarkar, Mahesh & Thakare, Swapnil & Surushe, Tanaji & Giri, Amol & Chopade, Vaibhav. (2021). Formulation and Evaluation of Liposome by Thin Film Hydration Method. Journal of Drug Delivery and Therapeutics. 11. 72-76. 10.22270/jddt.v11i1.4677.
3 https://www.fda.gov/media/70837/download
NANOPARTICLE KNOWLEDGE
Looking to learn more about nanoparticle ? Do you need inspiration or a different angle to take on your specific problem? Well, we probably have an application note just for you, feel free to check them out!
Endosomal Escape: A Critical Challenge in LNP-Mediated Therapeutics
Endosomal escape of lipid nanoparticles (LNPs) is a crucial process in the intracellular delivery of mRNA-based therapies and vaccines. After cellular uptake via endocytosis, LNPs must efficiently release their mRNA payload from endosomes into the cytoplasm to ensure proper translation into therapeutic proteins. This step is vital for the success of LNP-mediated delivery systems, as…
Introduction to PLGA Nanoparticles as a Drug Delivery System
For the past decades, modern medicine shifted more and more towards the use of nanocarriers as drug delivery systems (DDS) to overcome the inherent drawbacks of traditional drug administration. To that end, several drug carriers were developed, ranging from lipid nanoparticles (LNPs), and solid nanoparticles (SLNs), to polymersomes, liposomes, and polymeric nanoparticles. On the latter,…
The Evolution of Nanomedicine: from ideas to clinical applications
Nanomedicine represents a transformative approach to diagnostics and therapeutics, leveraging the unique properties of materials at the nanoscale. Its roots extend back to the mid-20th century when the concept of using nanometer-sized particles for medical applications began to materialize. The field has grown significantly over the decades, offering novel solutions in drug delivery, imaging, and…