mRNA-LNP vaccines
With more than 5.5 billion people vaccinated worldwide during the Covid-19 pandemic, mRNA vaccines have come up as a highly effective strategy to fight quickly emerging diseases.
This unique capability to provide a quick, safe, and efficient therapy encouraged all the major players in the pharmaceutical industry to explore their use for a wide array of diseases including HIV, flu, Lyme diseases as well as cancer. Through this review, we get an in-depth introduction to their working principle, before exploring the two areas where they are the most promising: infectious disease and cancer.
What is a vaccine?
Vaccines were discovered more than 200 years ago and have ever since proven incredible efficiency in preventing the spread of infectious diseases, with more than 2 million deaths prevented each year. There are two types of vaccines: prophylactics, designed to prevent future pathogen infections, and therapeutics, intended to combat diseases that have already occurred, each with a different level of immunity.
Conventional ones typically contain inactivated, attenuated, or dead organisms, used to reduce the risk of illness by triggering an immune response. However, those conventional approaches have major disadvantages that limit their application in disease prevention and treatment:
1/ Their fabrication are usually lengthy and requires labor-intensive and time-consuming processes
2/ Their yield is generally very low
3/ They can lead to large risks of infection through contamination
In addition to this, they cannot address all kinds of diseases and are generally used only for diseases that can be grown in culture. It is to overcome these limitations, which would not allow for a quick worldwide roll-out, that innovative mRNA vaccines were used during the Covid19 pandemic.
Introduction to mRNA vaccines
Messenger RNA (mRNA) vaccines are based on the intracellular delivery of a single nucleotide sequence, which is then translated into protein, eventually using the body's own cells for inducing an immune response.
In practice, the strand is encapsulated into a lipid nanoparticle (LNP) - a sub-micro particle specially engineered to encapsulate and deliver nucleic acid to the targeted cell or organ - to form an LNP-mRNA complex. Once injected into the body, this complex binds to the target cell, either passively or via active targeting, before it gets internalized by the cell through the endocytosis process and released within the cell via the endosomal escape . The drop of pH occurring at the late stage of endocytosis leads to the collapsing of the complex, liberating the trapped RNA strand into the cytoplasm. Once in the cytoplasm, the mRNA strand will bind to a ribosome, before being translated into a protein, which will then trigger the immune system response. Check our review on intracellular mRNA delivery via lipid nanoparticle to learn more.
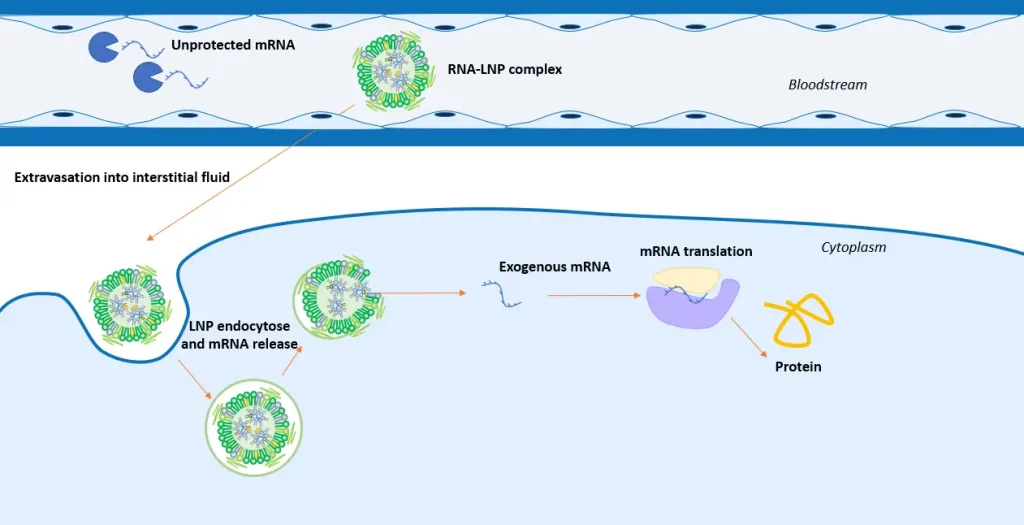
Main advantages
Considering its working principle, this type of vaccine offers major advantages compared to conventional ones in multiple aspects:
- They allow for the manufacturing of proteins that cannot be produced in bioreactors
- They avoid any potential risk of interference with the host genome (unlike DNA vaccines)
- They are an inherent cell-free process, making it scalable and cost-effective
Drawbacks
As every rose has its thorns, current RNA-based vaccines also have drawbacks compared to conventional ones such as:
- Poor stability
- Low-temperature conservation
- Potential immunogenicity
However, most of these hurdles can be overcome by optimizing the delivery vessel: the lipid nanoparticle (LNP). For all these reasons, mRNA-LNPs have become a promising tool for the treatment of numerous diseases.
mRNA-LNP vaccines manufacturing
The multiple-step process used to produce those vaccines are illustrated in Figure 2:
1/ It starts with the design of the complementary sequence of the targeted protein, surrounded by its head and tail (start codon, stop codon, 5’ cap, 5’ UTR…) simulating an endogenous mRNA structure to maximize its gene expression and improving its stability.
2/ Once identified, the target mRNA sequence can be manufactured through 3 main methods:
- Chemical Synthesis: This is the simplest, most continuous, and cost-effective approach. It is suitable for short RNAs (<100 nucleotides) but is not practical for longer oligonucleotides due to limitations.
- Host Cells: Recombinant production using host cells (e.g., E. coli) remains challenging to scale up for large-scale manufacturing, despite recent scientific advancements aimed at preventing RNA degradation.
- Enzymatic Synthesis: Modern RNA synthesis is increasingly exploring two enzymatic synthesis methods: Polymerase Chain Reaction (PCR) coupled with Polymerase Chain Transcription (PCT) and advanced in vitro transcription (IVT).
As PCT still requires further improvements, especially in terms of scaling up production, IVT has naturally emerged as the current industry standard for large scale manufacturing. In this process, a DNA template encoding the desired protein is transcribed into mRNA using an enzyme.

3/ The synthesized strands are then purified by dialysis, or Tangential Flow Focusing (TFF).
4/ The RNA strand should now be encapsulated into a lipid nanoparticle to optimize its delivery, forming the mRNA-LNP complex.
LNP were traditionally produced using batch processes and high-energy techniques such as thin film hydration, high-pressure homogenization, and sonication. However, these methods exhibited significant drawbacks in terms of consistency and yield, rendering them unsuitable for vaccine development.
As a result, the LNP production has transitioned to self-assembly methods. In this approach, a solvent, typically ethanol in which lipids are dissolved, is combined with an antisolvent, typically low pH aqueous buffer containing the RNA strand.
During the mixing process, these two suspensions merge, which causes a decrease in the solubility of the lipids in the medium and triggers the spontaneous self-assembly of the mRNA-LNP complex.
Given the critical influence of mixing conditions on the quality attributes of the LNP (size, PDI…) microfluidics has emerged as the most widely used technique for manufacturing mRNA-LNPs. It indeed offers the unique ability to precisely replicate mixing conditions while being scalable from the first steps of the drug development process (µL) to production (L) making it the gold standard method for specialists in the field.
5/ Once formed, the mRNA-LNP suspension is purified and strerilized before being injected into the host body.
Unlock the next-generation
RNA-LNP vaccines with our
nanoparticles manufacturing
platform
mRNA vaccines applications

Infectious diseases
Preventing infectious diseases is the application for which this novel generation of vaccines is currently the most widely used. When used for infectious diseases, mRNA sequences are designed to induce an antiviral immobility by triggering the immune system
Numerous trials have already been conducted on various diseases. The most advanced ones are summarized in the side table, as of October 2023:
The example of Covid-19
Since the beginning of 2021, the world has grappled with the severe acute respiratory syndrome coronavirus 2 (SARS-CoV-2) pandemic, causing countless infections and millions of deaths. While many cases are mild, severe infections can trigger uncontrolled immune responses in the lungs, leading to pulmonary damage and edema.
While developing the COVID-19 vaccine, scientists identified that the spike protein on the surface of the SARS-CoV-2 virus facilitates its entry into host cells. For this reason, the spike protein represents a prime target for SARS-CoV-2 vaccines encoding either the receptor-binding domain or the full-length spike protein. The two mRNA vaccines currently approved for widespread used (Moderna's Spikevax® and Pfizer/BioNTech's BNT162b2) used this approach. The mRNA strand was then encapsulated within a lipid nanoparticle for an efficient intracellular delivery while minimizing potential toxicity and side effects (see the detailed lipids nanoparticle composition of the COVID19 vaccine)
Phase I trials of Spikevax®, showed safety and efficacy. It triggered robust humoral and cellular immunity against SARS-CoV-2, providing protection in preclinical models. The subsequent phase I trial in humans demonstrated a strong humoral immune response with mild-to-moderate adverse events. Phase III trials confirmed Spikevax®’s effectiveness, with a 94.1% reduction in SARS-CoV-2 infection and 100% protection against severe COVID-19. Subsequent studies compared Spikevax® and BNT162b2, with the former showing an advantage in terms of lower breakthrough infection rates and hospitalizations.
To combat the evolving Omicron variant, booster doses of vaccines have been authorized. Pfizer-BioNTech's and Moderna's updated booster shots contain bivalent formulations with elements from the original virus and the Omicron variant. These boosters have demonstrated efficacy in enhancing immune responses against Omicron, providing a strategy against emerging variants.
Yet, while mRNA vaccines have been instrumental in the fight against COVID-19, challenges remain. Questions about the duration of protection, boosting strategies, and minimizing adverse effects persist. Long-term monitoring and ongoing research will provide valuable insights to ensure the safe and effective use of mRNA vaccines not only against COVID-19 but also for other developments.
mRNA vaccines for cancer
In the context of cancer, those vaccines are usually used in therapeutic approach rather than prophylactic. Indeed, instead of looking to induce an antiviral immunity by triggering the immune system, mRNA sequences are here designed to activate antitumor immune responses by encoding for tumor-associated antigens (TAAs) or neoantigens . This flexibility allows it to target a wide range of cancer types including Melanoma, breast, brain, ovarian, pancreatic cancer.
As for infectious diseases, tens of trials are currently ongoing for cancer vaccines, here is a list of the most advanced ones:

The example of Melanoma cancer
Melanoma is a cancer that originates from the malignant conversion of melanocytes, which are found throughout the body, including the skin, mucosa, uvea, inner ear, and rectum. Cutaneous melanoma, the most common type, accounts for about 1.7% of all newly diagnosed primary malignancies and 0.7% of global cancer-related deaths.
mRNA vaccines, particularly those based on dendritic cells (DCs), have been investigated for melanoma treatment. Recent research involves numerous DC-based mRNA vaccines for melanoma patients, aiming to induce immune responses against various tumor-associated antigens (TAAs). TriMix, an mRNA vaccine, has been used in clinical trials due to its immune-stimulating components. Combined with immune checkpoint inhibitors like ipilimumab, it has shown encouraging results in advanced melanoma. Additionally, in vitro transcription-based vaccines, such as BNT111 by BioNTech, have been tested in clinical trials, demonstrating safety and immune responses.
Personalized vaccines targeting patient-specific mutations have shown potential, with some patients experiencing complete responses. Further research and combination therapies may enhance the effectiveness of mRNA vaccines for melanoma treatment, although no official approvals have been granted yet.
Other applications
In addition to this, mRNA vaccines have also been investigated for use in numerous fields. Thanks to their versatility, they can address a wide variety of diseases including treatment of autoimmune diseases, tissue damage, or more generally rare diseases, for which low prevalence generally limits their understanding.
Conclusion
mRNA vaccines have gained prominence in disease prevention and treatment, particularly in infectious diseases and cancer, but their widespread application faces several challenges.
Achieving a balance between designing and synthesizing the optimal RNA strand for the application, optimizing the LNP vessel for efficient delivery, and bringing them to production is challenging as large-scale manufacturing is currently hindered by the lack of continuous processes.
Strict temperature requirements for storage and transportation limit their distribution, and concerns about safety, including rare adverse events, persist. However, their unique ability to provide a quick go-to production for small-volume batches would open many doors to personalized medicine.
Looking to improve your RNA vaccine delivery efficiency ? Reach out to us!
NANOPARTICLE KNOWLEDGE
Looking to learn more about nanoparticle ? Do you need inspiration or a different angle to take on your specific problem? Well, we probably have an application note just for you, feel free to check them out!
Endosomal Escape: A Critical Challenge in LNP-Mediated Therapeutics
Endosomal escape of lipid nanoparticles (LNPs) is a crucial process in the intracellular delivery of mRNA-based therapies and vaccines. After cellular uptake via endocytosis, LNPs must efficiently release their mRNA payload from endosomes into the cytoplasm to ensure proper translation into therapeutic proteins. This step is vital for the success of LNP-mediated delivery systems, as…
Introduction to PLGA Nanoparticles as a Drug Delivery System
For the past decades, modern medicine shifted more and more towards the use of nanocarriers as drug delivery systems (DDS) to overcome the inherent drawbacks of traditional drug administration. To that end, several drug carriers were developed, ranging from lipid nanoparticles (LNPs), and solid nanoparticles (SLNs), to polymersomes, liposomes, and polymeric nanoparticles. On the latter,…
The Evolution of Nanomedicine: from ideas to clinical applications
Nanomedicine represents a transformative approach to diagnostics and therapeutics, leveraging the unique properties of materials at the nanoscale. Its roots extend back to the mid-20th century when the concept of using nanometer-sized particles for medical applications began to materialize. The field has grown significantly over the decades, offering novel solutions in drug delivery, imaging, and…