Exploring LNP Size and its Significance in Drug Delivery

Introduction
The development of advanced drug delivery systems, such as lipid nanoparticles (LNP) has revolutionized modern medicine. These sub-micron carriers, composed of a combination of lipids, offer a versatile platform for transporting an array of therapeutic agents: mRNA, siRNA, small drugs... A critical factor in optimizing their performance is the width of these nanoparticles which plays a pivotal role in determining their behavior within biological systems, influencing their efficacy, stability, and cellular uptake. In this article, we will explain the influence of lipid nanoparticle size on the drug delivery process, introduce you to the methods to measure it accurately and elaborate on how you can tailor LNPs for an optimized delivery efficiency.
Why is Lipid Nanoparticle Size Important?
The size of lipid nanoparticles is a critical attribute that significantly affects their performance as drug delivery carriers. In practice, Stability, encapsulation efficiency, drug release profile, bio-distribution, and cellular uptake are amongst the most affected delivery parameters influenced by its size.
Impact on Biodistribution
Smaller lipid nanoparticles tend to exhibit both prolonged circulation times and an enhanced ability to accumulate at target tissues. Tissue accumulation is principally due to a phenomenon known as the enhanced permeability and retention (EPR) effect, in which small nanoparicles leak through blood vessels and evade immune surveillance to accumulate around tumors. Learn more about it in our nanoparticle targeting review. Additionally, prolonged circulation time can be obtained by using nanoparticles between 20 and 150 nm, which helps reduce liver clearance and kidney filtration. [1]
Importance for Cellular uptake
The diameter of lipid nanoparticles also plays a decisive role in determining their cellular uptake and internalization. The most important cellular uptake mechanism for small molecules is endocytosis. Endocytosis relies on the transport of material into the cells by enveloping them in its phospholipid bilayer. Numerous endocytosis mechanisms are reported, and the kinetic of entry will differ depending on the nanoparticle size. At around 100 nm, RNA-LNP complex will be mostly taken up through receptor-mediated endocytosis, which also applies to viruses such as influenza (80nm) and reoviruses (120nm) [2]
Additionally, it is important to take into account that ideal particle characteristics – such as lipid nanoparticle surface chemistry - would vary from species to species: For instance, optimal RNA-LNP size for non-human primates appears to be smaller (50-60nm) than for rodents (70-80nm) [3]
In summary, the process of cellular uptake through endocytosis is significantly impacted by the LNP physicochemical characteristics of nanocarrier systems, where particle size and polydispersity index (PDI) play key roles. Read more on the endocytosis and endosomal escape process in our review.
Lipid Nanoparticle Size Characterization Methods
As we previously introduced, it is a key factor for efficient nanoparticle delivery, consequently, accurate characterization of LNP population is imperative for designing effective drug delivery systems.
Nanoparticle diameter definition
First, it is important to note that several definitions are available for nanoparticle diameter, with the 2 most commonly used ones being: Hydrodynamic diameter or Core diameter.
Hydrodynamic diameter
The hydrodynamic diameter of a particle represents the diameter of a solid sphere that encounters an equivalent drag force to that experienced by the given particle as it moves through a liquid.
This parameter is important in the context of lipid nanoparticles as those are generally surrounded by a PEG brush structure (see our review on LNP for more information on the importance of PEG). Considering this, using hydrodynamic diameter to compute payload volume can lead to significant errors as the actual dimensions of the nanoparticle containing the payload would be smaller.

Core diameter
Oppositely, the core diameter only takes into account the width of the inner core of the nanoparticle.
In the previous example, this would be the value to use to compute an actual LNP payload volume.
LNP size measurement tools
A range of techniques are employed to determine this critical parameter, each offering specific insights into the physical characteristics of these nanoparticles. Here is an introduction to the most used methods for lipid nanoparticle size measurement:
Dynamic light scattering (DLS) for hydrodynamic diameter measurement
DLS is the most commonly used technique for lipid nanoparticles size characterization. It works by measuring the temporal fluctuations of scattered light due to Brownian motion, providing information about the hydrodynamic diameter of LNPs in solution.
In practice, a polarized laser light source will shoot light through a sample, where it will be diffracted. The scattered light will be measured by a photomultiplier and processed by an autocorrelator to obtain the variation of light intensity over time. Light going through a sample filled with small nanoparticles will show very dynamic measurements, while larger nanoparticles will show slower intensity changes. The final size distribution is then processed from these data.
While DLS is adept at revealing size distribution and polydispersity, it can occasionally overestimate it due to the presence of the solvation layer. The newest generation of DLS systems also allows for the measurement of Zeta potential, making it a very versatile tool for LNP characterization.
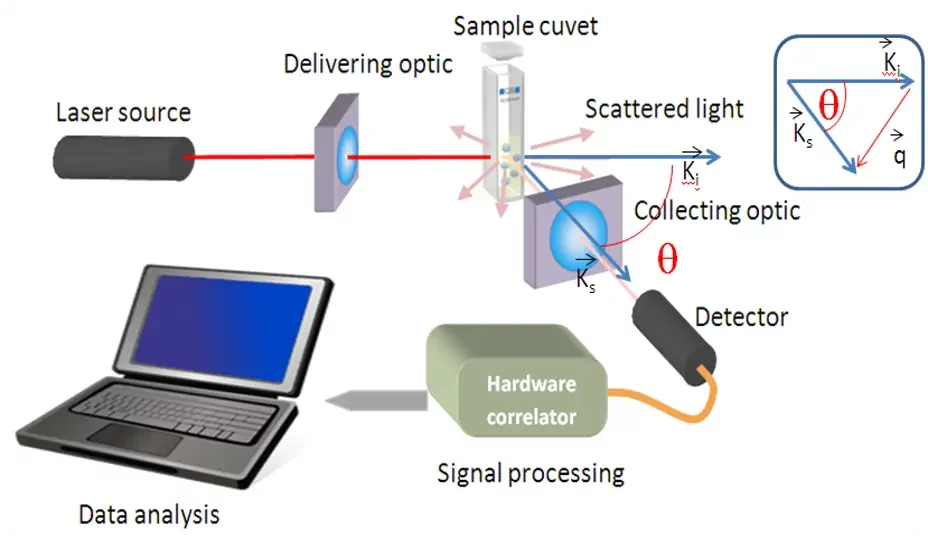
Cryogenic transmission electron microscopy (Cryo-TEM) for lipid nanoparticle core diameter measurement
Cryo-TEM presents another powerful approach. This method entails the rapid freezing of lipid nanoparticles to preserve their native structure, followed by imaging using transmission electron microscopy. Cryo-TEM furnishes high-resolution images that lay bare the morphology and diameter of LNPs – including both the hydrodynamic and core diameter.
However, it's worth noting that Cryo-TEM is slow, labor-intensive, and expensive, and can only be used to characterize a small part of the samples but not batches.
Tunable Resistive Pulse Sensing (TRPS)
TRPS operates based on the principle of electrophoresis, which involves applying an electric field across a nanopore embedded in a thin membrane that separates two chambers containing the sample and the electrolyte solution. The nanopore acts as a single-molecule sensor, allowing particles to pass through one at a time. As particles traverse the nanopore, they cause temporary changes in the electrical resistance, creating characteristic electrical pulses that are analyzed to extract valuable information about the particles, including size.
While providing numerous valuable information, TRPS can be tricky to use as it requires extremely precise calibration, and nanopore clogging can regularly occur in the system, requiring long sample preparation.
Additionally, other techniques such as static light scattering, electron microscopy, and atomic force microscopy contribute to the understanding of LNP volume and morphology.
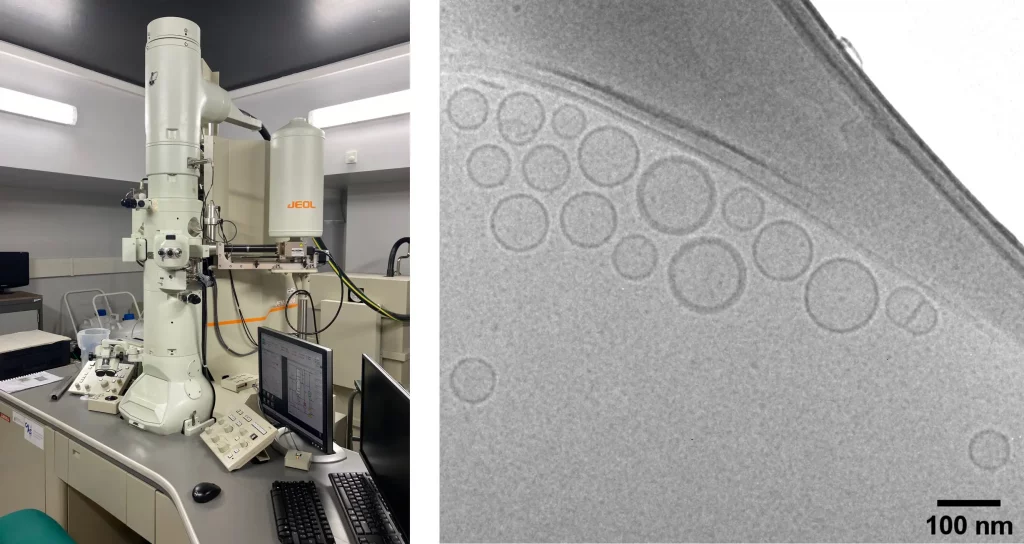
Nanoparticle tracking analysis (NTA)
Nanoparticle tracking analysis (NTA) operates on the principle of tracking individual nanoparticles' Brownian motion in real time. This technique offers insights into particle size, its distribution, and concentration, making it particularly well-suited for heterogeneous samples.
However, it is not well suited for nanoparticle with diameter smaller than 30 nm due to the influence of Brownian motion and thus requires good knowledge of the instrument to properly use it.
How to control Lipid nanoparticle size?
The critical characteristics of LNPs are influenced by a multitude of factors, stemming from both formulation and processing stages.
Influence of composition
The lipid nanoparticle composition stands as a pivotal factor, with the choice of lipids and their ratios intricately determining LNP physicochemical characteristics.
First, the helper sterol lipid content, influencing lipid packing and nanoparticle dimensions depending on the lipids it is linked to, will greatly impact LNP size and shape.
Additionally, PEG-lipid, though only present in a very small proportion, seems to play a major role on the final nanoparticle volume. In a study by M. Lokugamage et al. [4], it was demonstrated that lipid-based nanoparticles lacking PEG were notably larger, exceeding 200nm, in comparison to those containing as little as 0.5% mol PEG, which maintained a size of approximately 80nm. [5] In practice, lipid nanoparticle composition of commercially available vaccines generally contains around 1.5% of PEG-lipids.
This would be explained by the fact that the PEG-lipid is primarily situated at the surface of LNPs. Consequently, increasing the mol% of PEG-lipid (found on the surface) is believed to result in a heightened surface area-to-volume ratio, consequently leading to a reduction in particle dimensions.
It is also worth noticing, that counter intuitively, Lipid nanoparticle size is not influence by the size of the RNA cargo, as introduced in the study: A careful look at lipid nanoparticle characterization: analysis of benchmark formulations for encapsulation of RNA cargo size gradient
Influence of manufacturing process
The manufacturing methods are equally pivotal. Numerous techniques are available for the synthesis of lipidic nanoparticles.
Traditional methods like solvent evaporation or high-pressure homogenization tend to produce overall poor critical characteristics control due to erratic mixing mechanisms, leading to broad nanoparticles this limitationdistribution and very low reproducibility.
To overcome these limitation, novel synthesis techniques based on self-assembly have been developed. In those, two liquids: a solvent – usually ethanol containing the lipids - and an anti-solvent – usually water with dissolved RNA - are mixed, triggering the self-assembly of LNP. With these methods, the faster the mixing speed, the smaller the particles will get, allowing the user to finely tune their nanoparticle size simply by playing with the synthesis parameters.
To obtain such a fine control of the mixing conditions, thus yielding more uniform LNPs population with much finer controlled sizes and improved repeatability, microfluidics mixing methods are used.
Microfluidics indeed offers the unique ability to produce repeatable and controllable mixing conditions, proving to be a major game changer for those applications.
Have a look at our microfluidics synthesis of nanoparticle review to learn more about the microfluidics synthesis of LNP!
On top of this, as introduced in our article on the influence of dialysis on LNP parameters, the post-process solvent extraction step can lead to major changes on the nanoparticle.
Further still, processing parameters such as mixing speed, temperature, concentration, and solvent choice directly impact LNP proportion during formulation. The physical stability of LNPs plays a role as well; events such as aggregation or fusion can alter their dimensions over time.
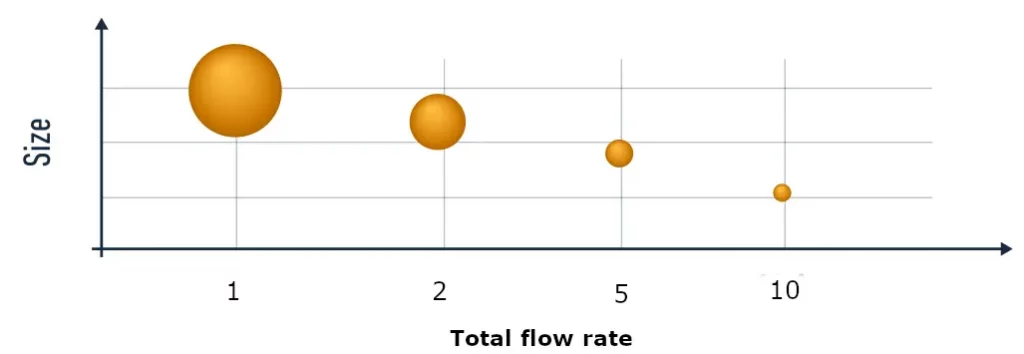
Conclusion
Lipid nanoparticles have reshaped the realm of drug delivery by offering a platform for targeted and controlled therapeutic agent release. Among the myriad of parameters steering LNP behavior, diameter emerges as a pivotal factor influencing biodistribution, cellular uptake, and overall effectiveness. Employing advanced characterization techniques like DLS, Cryo-TEM, and TNA, scientists gain insight into LNP size, allowing for tailored drug delivery systems. Additionally, in light of the importance of the composition and the manufacturing methods on the final nanoparticle critical characteristics, it appears to be extremely important to choose them very carefully.
In the dynamic field of nanomedicine, understanding the factors shaping RNA-LNP characteristics holds the key to precision drug delivery systems with enhanced therapeutic impact, for example in cancer drug delivery.
Interested in finer control of your nanoparticle size ?
Reach out to us to get access to the most advanced microfluidic-based nanoparticle synthesis systems
References:
[1] Di, Jinwei et al. “Size, shape, charge and "stealthy" surface: Carrier properties affect the drug circulation time in vivo.” Asian journal of pharmaceutical sciences vol. 16,4 (2021): 444-458. doi:10.1016/j.ajps.2020.07.005
[2] Hassett, Kimberly J., et al. "Impact of Lipid Nanoparticle Size on MRNA Vaccine Immunogenicity." Journal of Controlled Release, vol. 335, 2021, pp. 237-246, https://doi.org/10.1016/j.jconrel.2021.05.021. Accessed 24 Aug. 2023.
[3] Lam, Kieu, et al. "Optimizing Lipid Nanoparticles for Delivery in Primates." Advanced Materials, vol. 35, no. 26, 2023, p. 2211420, https://doi.org/10.1002/adma.202211420. Accessed 24 Aug. 2023.
[4] https://nanoparticleanalyzer.com/blog032721.php#:~:text=The%20hydrodynamic%20diameter%20of%20a,as%20the%20particle%20in%20question.
[5] Lokugamage, Melissa P et al. “Optimization of lipid nanoparticles for the delivery of nebulized therapeutic mRNA to the lungs.” Nature biomedical engineering vol. 5,9 (2021): 1059-1068. doi:10.1038/s41551-021-00786-x